
Quantum theory first appeared in the early part of the 20th Century and was used to explain how light and matter behave at a fundamental level. Today, a new generation of quantum technologies has been created through engineering and exploitation of naturally occurring quantum effects.
Quantum theory has fundamentally changed our understanding about how light and matter behave at extremely small scales. In this century, the possibilities of using quantum effects in many systems lead us to the so-called second quantum revolution, in which sensors and measurement devices will be developed, even of smaller size, but with higher performance and measurement capacity, together with quantum computers that will overcome the current importance of Information and Communication Technology (ICT)).
The applications of these technologies will reach all sectors and, like any revolution, will require, already requires, a quantum metrology that develops high resolution and accuracy methods for the measurement of physical quantities based on quantum mechanics, particularly through the so-called quantum entanglement. Classical measurements are limited by statistical and systematic errors. Statistical errors, originating either from insufficient control of the measurement system or from physical limits such as the Heisenberg uncertainty principle, can be reduced by repeating the measurement n times and then averaging the outcomes. This process converges to a Gaussian distribution with a standard deviation that scales as n−1/2. However, using quantum effects such as entanglement and squeezing makes it possible to improve measurement precision beyond this level, decreasing the error by an amount proportional to n−1.
Light has played a key role in metrology since 1960, when the metre was defined by the number of vacuum wavelengths of a particular spectral line of 86Kr. More recently, optical metrology has not only seen significant improvements in precision for such tasks, but has also greatly extended its use to size measurements of subatomic particles. Two of the most useful tools developed within this field so far are perhaps the optical frequency comb and the optical clock, both tools have significantly improved our ability to make precise measurements of frequency and time.
Optical metrology is also being used to measure ultraweak forces, which is a growing requirement in the field of nanotechnology. One challenge in particular is the measurement of the Casimir interaction, a submicrometre-scale force induced by quantum fluctuations of the electromagnetic field between two neutral bodies. Over the past ten years, dramatic progress has been made in the theoretical understanding and measurement of the Casimir force. New computational methods now allow us to analyse the Casimir force between both planar and non-planar geometries
An important step in the use of quantum theories to metrology is the revision of the international system of units, the SI, scheduled for October next year, which will be defined around the numerical values of seven constants of nature, and which will allow the practical realization of all the units through physical experiments in which quantum phenomena play a crucial role.
In this field, the Institute of Quantum Optics and Quantum Information (IQOQI) of the Austrian Academy of Sciences devotes its work to basic theoretical and experimental research in the areas of quantum optics and quantum information. The research topics include since the basics of quantum physics up to its applications, for example, in metrology, sensor technology or quantum information processing.
Experimental physicist Rainer Blatt, Research Director of IQOQI has carried out trail-blazing experiments in the fields of precision spectroscopy, quantum metrology and quantum information processing. He works with atoms caught in ion traps which he manipulates using laser beams. This work is based on suggestions made in the mid1990s by theorists Ignacio Cirac and Peter Zoller. In 2004, using their suggested set-up, Blatt’s working group succeeded for the first time in transferring the quantum information of one atom in a totally controlled manner onto another atom (teleportation). The science journal Nature reported the experiment and gave it pride of place on the cover. Two years later, Rainer Blatt’s working group already managed to entangle up to eight atoms in a controlled manner. Creating such a first “quantum byte” (qubyte) was a further step on the way towards a quantum computer. In 2011 the team managed to push this record to 14 entangled atoms. Furthermore Rainer Blatt took important steps towards successful quantum error correction and the building of quantum simulators.
For e-medida it is an honor to be able to interview Dr. Blatt, and ask him directly about interesting questions such as those outlined in this brief introduction.
To start with this interview one general key question that is not new for you: Is quantum technology the future of the 21st century?. If so, what problems in this field, either technical or theoretical, would you most like to see solved?
There are many new quantum technologies now available, which are beyond those that changed the world in the so-called 1st quantum revolution. Since the mid 20s of the last century, we have learned the fundamentals of quantum physics, and how to use it for many applications, ranging from controlling radiation in masers and lasers over the production of electronic elements to medical imaging and the modern GPS. Beyond that, over the last few decades we have learned to create and manipulate entanglement to enhance existing quantum technologies and to use it for new applications. Its applicability ranges from quantum communication to quantum metrology and sensing to quantum simulations and eventually to quantum computing. From all of these fields, the realization of a fully scalable quantum computer is among the most challenging and rewarding. If available, many very difficult problems could be tackled. While no theoretical roadblock seems to prevent us from getting there, it remains a very challenging task awaiting its realization. This is not only a technological or experimental problem, theoretical progress will help in future realizations and implementations.
What exactly does second quantum revolution mean?. Will the second quantum revolution only benefit highly developed countries or regions in the world that invest heavily in cuttingedge research?
As pointed out above, it is the use of entanglement for new experiments and devices, which will allow us to use technologies with capabilities way beyond what is available today. This defines the 2nd quantum revolution as opposed to what is available for many decades now.
As we have already seen over the last decades, successful developments can also happen in small(er) countries and eventually all applications resulting from the 2nd quantum revolution will benefit everybody. Clearly, the ability to build and further develop technical devices based on advanced quantum technologies rests on some availa ble infrastructure and certain investments in this area. However, this is not limited to highly developed countries. It rather depends on political and economic decisions whether countries and societies want to get involved with these technologies.
How do you see the development and the quantum level in Europe? In your opinion, during the next decade, what will be the main driver of quantum technology?
Quantum physics in Europe is well established and in fact, many of the developments leading to the 2nd quantum revolution were made in Europe first. Basic research and the quantum physics knowledge in Europe is cutting-edge and worldwide leading. Unfortunately, this is not the case when it comes to transferring these technologies to applications. Here, other parts in the world, in particular in the USA, are much more advanced and aggressive.
At this time, enormous investments are made in the US, in Canada, in Asia (especially in China) and in Australia to develop the new quantum technologies into industrial applications. Europe has seen this development and has initiated the European flagship program on Quantum Technologies, in which a total of one billion Euros will be invested over the next decade to further develop quantum technologies towards industrial applications.
On the shorter timescale, the technology driver will be quantum communication technologies, then quantum metrology and sensing applications.. On a longer timescale, we will see quantum simulators and finally quantum computers as the drivers for new technologies.
What can you tell us about the internationalization of research in this field? Collaboration between groups. Countries more advanced in these issues.
Cutting-edge research is always international and successful groups have always collaborated on an international scale. For further developments this will be intensified. On the other hand, the more the technological development is driven by companies, the less will be available publicly. Countries that support research and invest early in this domain will have an advance when it comes to market corresponding products.

A key question for more than one country is: how could a country develop and create a viable ecosystem capable of supporting a fast growing quantum technology industry?
Countries with established industries, for example in communication technologies, have an advantage because some infrastructure and key personnel are available. In order to create, support and sustain a growing quantum technology industry, it will be necessary to educate students in the respective areas, especially in the interdisciplinary field that is currently developed: “Quantum engineering”. Moreover, research institutes at universities and at national laboratories need to establish groups that are able to perform cutting-edge research to compete internationally.
What do you know about what is being done right now in Spain and Hispano America on these issues?
I know very little about the situation in these countries. Generally, basic research, mostly on the theory side, is well established in these countries, however, experimental research lacks the infrastructure and the financial backing to be able to catch up and compete at this time.
What working groups do you know?
Many very good groups and too many to be listed here.
How do you see the capacity of our R & D to face these challenges?
The quantum physics basis is there and as said above, the expertise is usually available, however, mostly on the theory side. Experimental efforts are available (in some areas). Thus, the scientific capacity is there. Meeting future challenges in quantum technologies however will require further investments in experimental infrastructure.
As you know, there are Research programmes in some world regions like EUROPE Ex.: EMPIR programme or Quantum flagship that are supporting the developments of quantum technology in order to be leader in the market. In your opinion, how long will it take them to produce marketable applications and products?. And more important what far-ranging changes to society and economics do you expect from the results of this research?
As the European flagship program clearly states: There is still a gap between academic research and the wide applications of quantum technologies. The question can therefore not be answered in simple terms. First, it depends on the respective technologies. For example, quantum communication technologies are sufficiently mature and marketable technologies; they are already available and/or will be made available in the next few years. On the other a hand, quantum computers for a more general use will become only available after further technological developments. For the programs to be efficient, it is required that (academic or other) research institutes work closely together with industrial partners.
The far-ranging changes to society and economics are enormous and could not possibly be summarized here. As an example, consider the development of the laser in 1960, consider its impact on todays technology and think about the changes it has brought since then. Quantum technologies will even have a wider impact.
Every industrial revolution has needed metrology as a necessary support for its development. In this quantum revolution, is a quantum metrology also necessary?
As pointed out, every technology needs intense research and support to be sustainable. Standards labs, which are usually the national metrology labs, define, observe and guarantee the standards; they calibrate devices to certify and ensure quality standards. Therefore, for the 2nd quantum revolution, it will be imperative to have such standards and therefore the respective metrology labs.
If so, what are the most critical and necessary metrological aspects for the development of this quantum revolution? What contemporary metrological field you think it should devote more resources and effort related to quantum technology?
For the quantum technologies being developed in the four areas focused on by the flagship program, we need standards in various ways. First and foremost, with the new definition of the fundamental constants based on quantum physics, quantum metrology on the respective fields is imperative. Special consideration is given here to atomic clocks, which provide the most precise tool today for all measurements.
For the quantum technologies being developed in the four areas focused on by the flagship program, we need standards in various ways. First and foremost, with the new definition of the fundamental constants based on quantum physics, quantum metrology on the respective fields is imperative. Special consideration is given here to atomic clocks, which provide the most precise tool today for all measurements.
For quantum simulations and quantum computations, standard tests for verification and validation have to be provided. This ensures that devices can be compared and evaluated across platforms. This is very similar to all classical metrological devices, for which the standards labs usually provide the metrology service.
Standards labs, define, observe and guarantee the standards, they calibrate devices to certify and ensure quality standards. Therefore, for the 2nd quantum revolution, it will be imperative to have such standards and therefore the respective metrology labs.

Quantum metrology research promises approaches to build new sensors that achieve the ultimate level of precision measurement and perform fundamentally better than modern sensors. In small and medium National Metrology Institutes we worry about how to focus on this next generation of the metrology in relation to the needs of development of new standards and the need or not of traceability of these new sensors.
I am personally not very familiar with the notion of traceability. Quantum enhanced sensors per se offer a better precision or better signal-to-noise ratios; however, they will need in most cases still a calibration.
Can you give us your opinions and some advises about how to face this challenge and design an overall strategy to be developed by the national metrology institutes?
Metrology institutes need to gather and to make sure that they have a common understanding of what their role should be in making sure that they can validate, verify and eventually calibrate quantum devices, such that industry knows the rules under which they can manufacture and finally sell their quantum machines in a fair and competitive way.
At a time of economic hardship, where many States are tightening budgets and prioritizing certain strategic lines, what actions would you recommend to address the goverment in relation to metrology and particularly with regard to the development of quantum technologies?
Simply, go finance it and invest in the development of such technologies. Make sure, your educational system is up for it, such that there are well enough educated people who can deal with the challenges. Otherwise, you are going to lose.
As you know, the SI is now being redefined based on constants of nature and their realization by quantum standards. Therefore, the underlying physics and technologies will receive increasing interest, and not only in the metrology community but in all fields of science. What can you tell us about the new definitions of basic units of measurement and the new SI?. In your opinion, will it affect significantly the development of science?
The redefinition of the fundamental constants was long planned for and is now timely since it bases every measurement on constants of nature and not any more on artefacts. This ensures a higher precision in the future and more and better reproducibility. Thus, it will be helpful for future development of science, which will further focus on the fundamental constants and the basic interactions in nature.
To finish this interview, could you tell us in what experiments is your research group currently working? Which difficulties do you foresee and which will need to be overcome?
My research group is concentrating on research concerning quantum optics and quantum information with trapped ions. In quantum optics we investigate the interaction of single photons with single atoms at a fundamental level; for quantum information purposes, we use strings of trapped ions as quantum bits, which are manipulated with focused laser beams. With such a device, we have built two quantum computers, which are currently used for quantum information processing with 14 and 20 qubits, respectively, as well as for quantum simulations. For future quantum networking, we have developed an interface with which we can transfer the quantum information stored in an atomic qubit to a photonic qubit, using a cavity QED experiment. For quantum metrology, we use a single trapped Al+ ion as a frequency reference, which is optically cooled and measured by quantum logic spectroscopy, using a Ca+ ion confined in the same trap. All in all, our research efforts are centred around basic research in the corresponding fields and the development of quantum technologies for all the relevant fields of future applications.
What is the importance for the future of these works and possible achievements?
As mentioned above, we are carrying out basic research at an academic level for both the development of new technologies and the education of a future generation of quantum physicists and engineers, who are then able to develop future applications. One of the best achievements that we could get would be the realization of a scalable quantum computer, which requires the implementation of quantum error correction to keep the qubits alive for the time of their employment (for quantum metrology, quantum simulations and quantum computations).
Uno de los mejores logros que podríamos obtener sería la realización de un ordenador cuántico escalable, lo que requiere la implementación de la corrección de errores cuánticos para mantener los qubits vivos durante todo su tiempo de utilización (en metrología cuántica, simulaciones cuánticas y computación cuántica).
Fields of application.
Mentioned above: quantum communication, quantum metrology (especially time and frequency standards), quantum simulators (analogue and digital) and eventually, quantum computation.
What about metrological applications?
All of fields above require precision measurements, in particular for time- and frequency standard applications. But also, quantum simulators (especially their verification and validation) require metrological work and, of course, quantum computers will have an enormous impact on metrology since they allow the full control of quantum coherences (at will), which allow for tailored states and quantum systems for all kind of specific measurements.
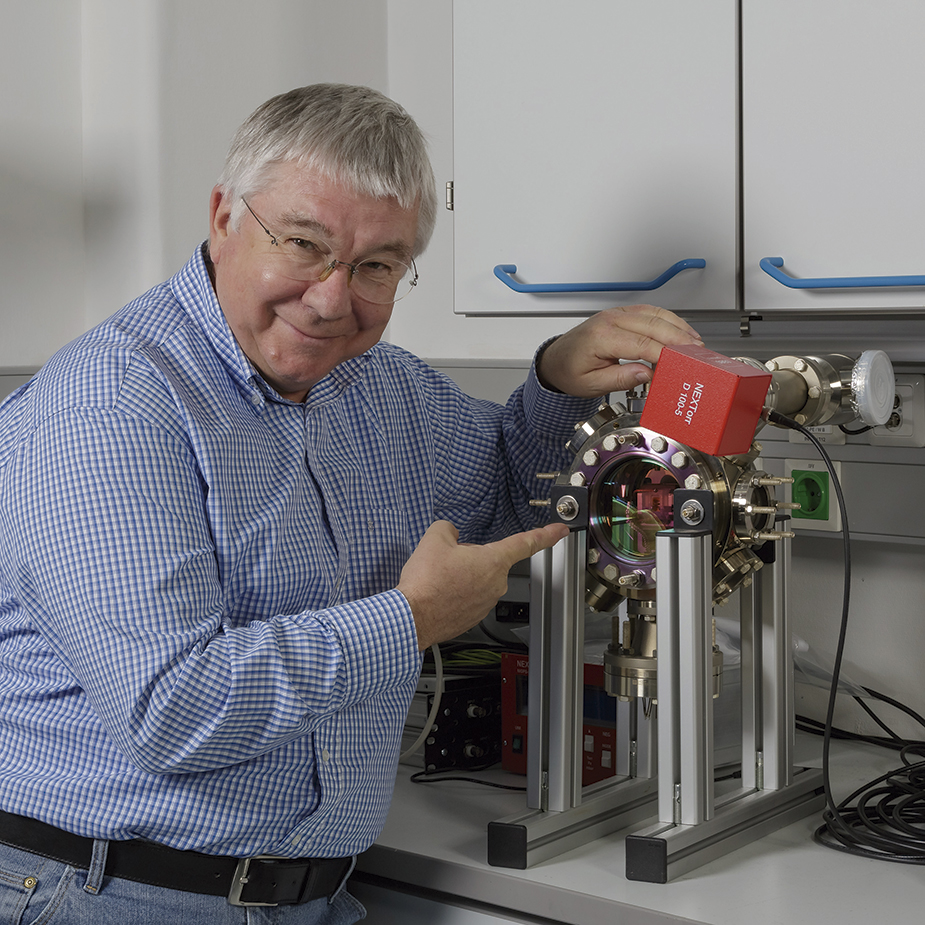
Prof. Blatt, we thank you for your kindness to the readers of e-medida and wish you the best in your current and future research.
e-medida is extremely grateful for the kindness of Prof. Blatt, and for the time and consideration which he has given us, including providing photos of great level and technological impact, included in this interview.
Also, e-medida thanks the time and support of Prof. Miguel Ángel Martin-Delgado, traveling to Innsbruck to make and get in person this interesting interview with Prof. Blatt.
Comentarios